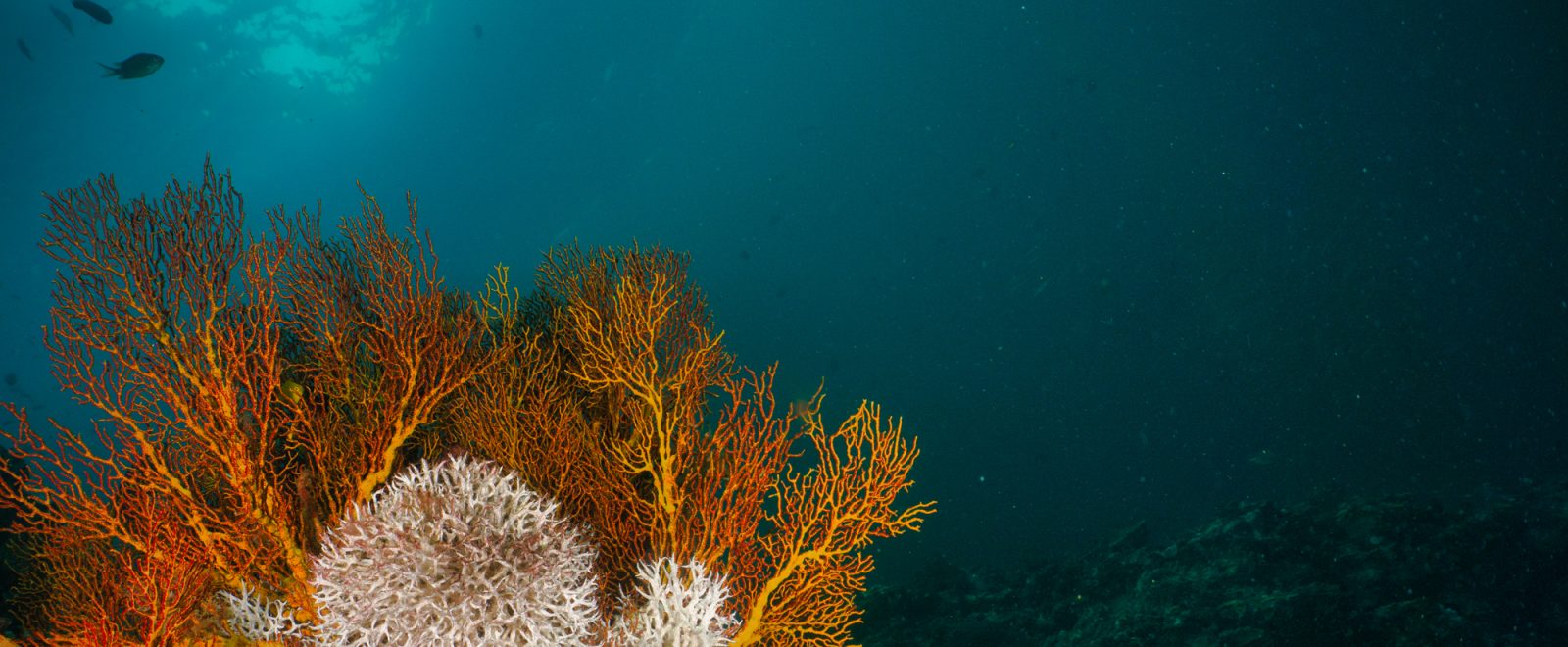
Hope for Coral Reefs
UW professor Pupa Gilbert believes these essential parts of Earth’s ecosystem are more resilient than we thought.
Four Hundred twenty-five million years ago, when Wisconsin was situated 20 degrees south of the equator, bathed in a shallow tropical sea, some of the first coral reefs began to take shape, mounded and rich in the ocean life of the Silurian Period. Trilobites, crinoids, and brachiopods swarmed the coral and left the fossil evidence of their existence as a record of the early marine environments of Earth.
The corals of the Silurian are long extinct. They were different from the corals we know today, but their fossils harbor the physical clues that show us they made their skeletons in precisely the same way as modern corals — and in almost exactly the same fashion as you and I and nearly every other animal make bones, teeth, and shell.
“All of these hard biominerals are actually made of crystals,” explains Pupa Gilbert, a University of Wisconsin–Madison physics professor and an expert among the small community of scientists seeking to understand how animals make nature’s hardest and most durable materials from scratch. “It seems completely bizarre to me that soft-tissue animals manage to form very hard crystalline tissue.”
Gilbert’s recent work on coral may prove to be especially salient. It is providing hints that the organisms that build the world’s great reefs have an innate resilience that may allow them to survive a major effect of climate change: increasingly acidic ocean waters caused by excess carbon dioxide in the atmosphere.
“Can reefs survive climate change?” Gilbert asks students in a lecture. “Yes, I think so, but others disagree.”
Knighthood
Gilbert joined the UW–Madison faculty in 1999. She is a pioneer in the use and development of spectromicroscopy using synchrotron light — bright beams of X-rays generated in specialized particle accelerators. Shining those X-rays on samples under souped-up microscopes is a way to illuminate the properties of materials at the most fundamental level. It yields pictures of the physical microstructures that tell us, for example, how human tooth enamel resists the grind of millions of chews, enough to last a lifetime — a finding Gilbert and her collaborators published in late 2019.
Gilbert came to UW–Madison after serving as a staff scientist at the Italian National Research Council and the Swiss Federal Institute of Technology in Lausanne. Before joining the Wisconsin faculty, she had been a frequent visitor to campus as a user of the university’s Synchrotron Radiation Center. With its particle accelerator, Aladdin, the center was then one of a handful of sources of synchrotron light for use in research worldwide. She was excited to come to Madison, recalls former UW chancellor and fellow physicist John Wiley MS’65, PhD’68, who helped recruit her to campus. “She’s quite a character. Very smart, creative, and clever. She’s always thinking.”
Gilbert’s early work had nothing to do with shells and bones. Her research centered on a treatment for glioblastoma, an incurable brain cancer, and it earned her a knighthood from her native Italy in 2001, as well as two patents through the Wisconsin Alumni Research Foundation.
One of Gilbert’s first offices on campus was in a cramped and drafty house trailer parked outside the now-decommissioned synchrotron amid the farm fields of rural Dane County near Stoughton. It was a favorite refuge for Gilbert not just because she was within a stone’s throw of the light source for her microscopy, but because she could smoke there. (She quit 12 years ago.)
Leaning into her subject in her Chamberlin Hall office, a space littered with biominerals — mother of pearl, mollusk shells, pieces of coral, shark teeth, fossils — she makes little effort to curb her enthusiasm for the subjects of her science.
“I came back with a passion for learning how organisms form crystals, how they deposit the hardest, stiffest, and toughest parts of their bodies, which in the case of humans are teeth and bones. For mollusks, it’s the shell. For corals, it’s the coral skeleton. I knew this was something that would entertain me for a lifetime.”
Gilbert’s curiosity and verve for science is cross-pollinated with a deep interest in things like cooking and art, which may help explain her fondness for biomaterials. Not only are they strong and tough, but biominerals can also be beautiful, as reflected in the iridescent sheen of mother of pearl and the honeycomb architecture of coral. (In 2012, her micrograph of the crystalline structure of a sea urchin tooth was a winner of the Science magazine and National Science Foundation’s International Science and Engineering Visualization Challenge.) Biomineral morphology, the myriad of shapes and sizes that serve an animal’s lifestyle needs — from the serrated tooth of a shark to the chambered spiral of a nautilus shell — is another draw.
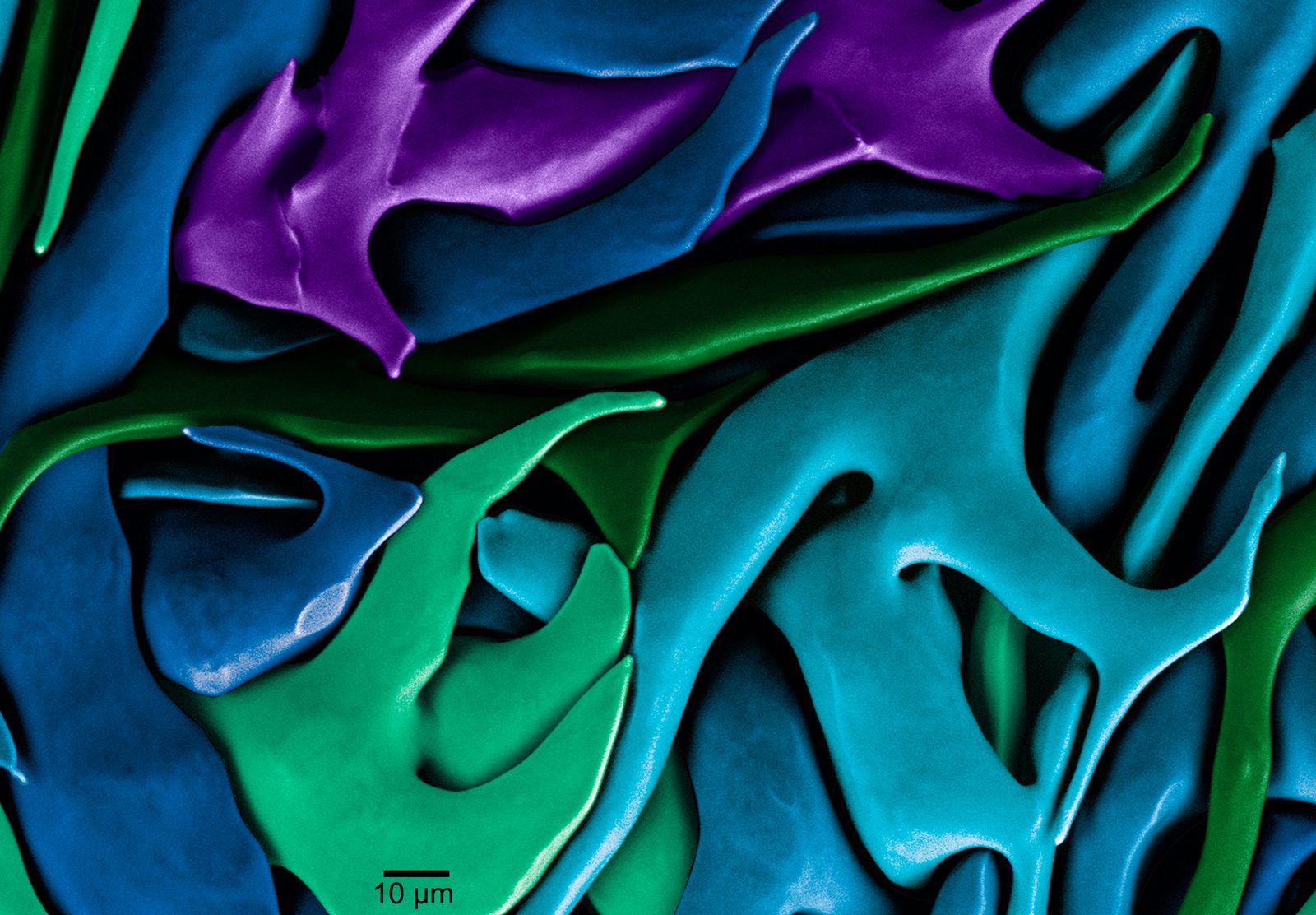
Gilbert’s work has been widely recognized, including with a 2018 Vilas Distinguished Achievement Professorship. She also received the top research award from the Advanced Light Source, the U.S. Department of Energy–funded synchrotron at the Lawrence Berkeley National Laboratory in California where Gilbert now conducts her research.
“For me, doing research is inescapable,” Gilbert says. “It’s like an itch I have to scratch. I can’t not do it.”
Seeing the Unseeable
Four or five times a year, Gilbert packs up her samples and her research group and travels to Berkeley to conduct experiments. Time on the synchrotron’s beamline is precious, and Gilbert’s experiments can take several days of round-the-clock work: “It’s a pain in the neck to have to travel to do your experiments. You have to fly your entire group. It’s very expensive. It’s complicated to get the beamtime to do the experiments, but it is well worth it. It is a fabulous synchrotron. It’s unique. No one else can do the experiments we do.”
Each experiment is planned like a moonshot. “We prepare like crazy. It is super hard emotionally and intellectually,” Gilbert explains. Intense effort goes into subjecting a sample of material — a fresh piece of coral, an abalone shell, a human tooth — to the wavelengths of synchrotron light that allow scientists to determine their structure and composition to the nanoscale, billionths of a meter.
The 12-hour shifts on the synchrotron’s beamline require exquisite planning, stamina, and keen attention to detail. “At the end of those long shifts, the relief and joy of success was something I got to experience,” says Kristin Bergmann, a professor of earth, atmospheric, and planetary sciences at MIT.
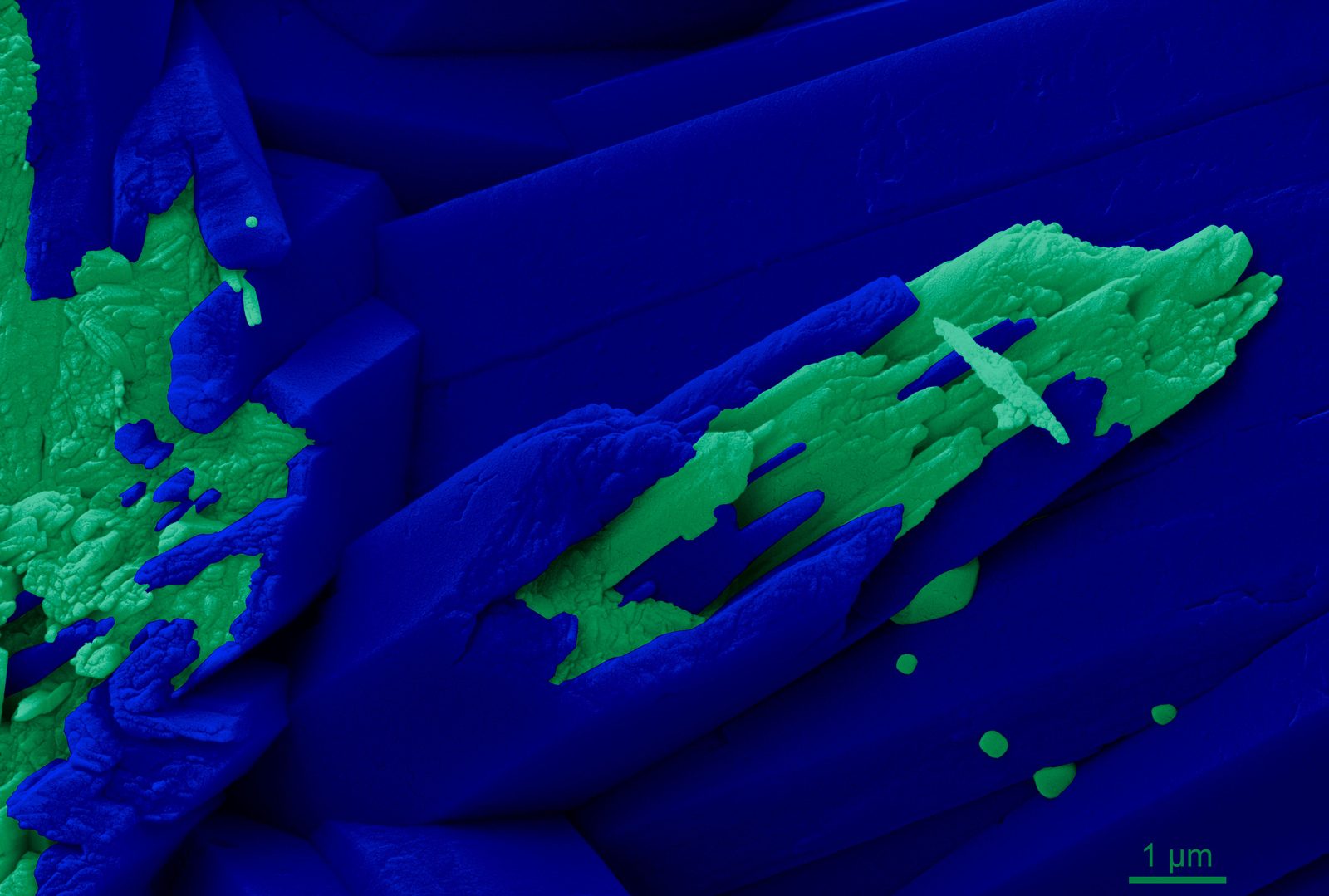
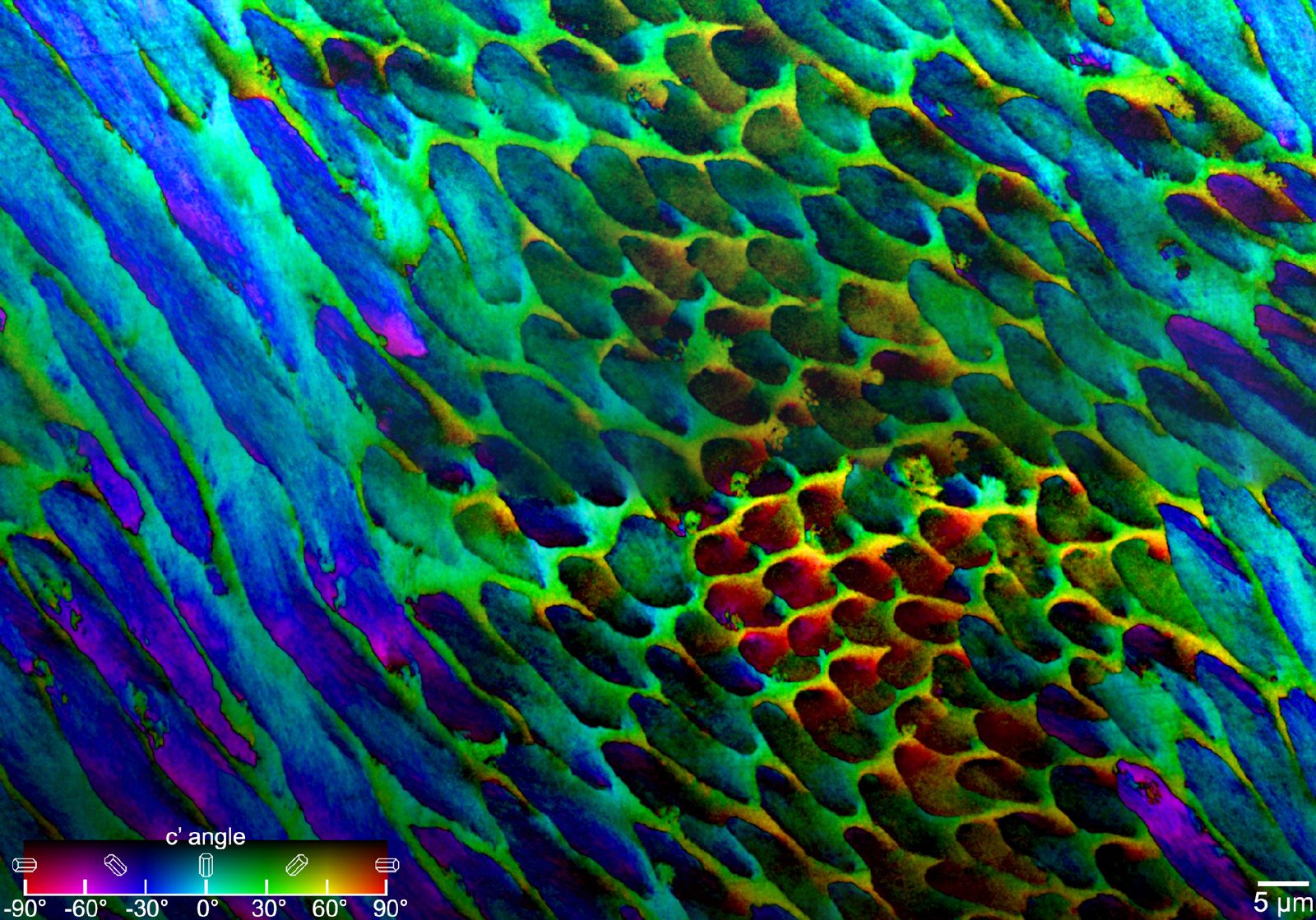
Bergmann first collaborated with Gilbert in 2014, when she was a postdoctoral fellow in the lab of Harvard professor Andrew Knoll, an expert on early life on Earth. Together, they were following up on Gilbert’s previous studies of clamshell microstructure, which suggested the microscopic polygonal tablets that compose a shell’s silky mother-of-pearl lining registered the temperature of the ocean water when the shells were formed. Applied to fossil shells the technique, Knoll explains, is a reliable method to take the temperature of oceans that existed hundreds of millions of years ago, useful insight for scientists reconstructing past environments.
Gilbert has found that several crystalline bio-minerals start as amorphous, which lets animals shape their skeleton or tooth or shell — like soft clay — to exact specifications. “They’re deposited as amorphous particles, so they can have any shape useful to the animal; then they slowly crystallize,” she says. The morphological instructions embedded in an animal’s genome give the structures “a very well-selected shape” that provides a function — be it shelter, locomotion, or aggression — the animal can use to give it a lifestyle edge.
Gilbert’s recent work on the skeletal building blocks of coral has led to a better understanding of precisely how coral polyps build skeletons that form the massive reefs that serve as oases in the deserts of the ocean floor.
Lead image by Placebo365/Getty Images. Additional photos by Jeff Miller.
Terry Devitt ’78 MA’85 is a veteran writer covering all aspects of science and technology. Haven broken several bones over the course of an eventful life, he was happy for the opportunity to better understand how skeletons and other hard structures are made by squishy life forms like people.
Published in the Spring 2021 issue.